LEADER: CHRISTINE KEATING
DESCRIPTION
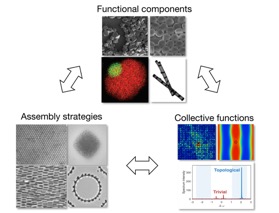
IRG4 Overview: Advances in materials synthesis and assembly, inspired by collective function goals, will enable new classes of reconfigurable photonic materials in which the optical response varies in response to particle reorganization.
IRG4 seeks to understand and control the organization of particles and particle mixtures to generate photonic architectures in which non-additive functions are imparted by the collective properties of the array. Assemblies will incorporate multiple, distinct particle populations that vary in composition and consequently in their response to various directed self-assembly approaches. Learning how to achieve desired assembly outcomes despite these differences, and to find ways to take advantage of them for increased control, will set the stage for a new era of nanomaterial-enabled device applications well beyond those proposed here. Several classes of multicomponent assemblies are under investigation, incorporating new types of functional particles and spanning a wide range of organizational ordering schemes. These span a range of organizational complexity from well-ordered arrays that will collectively define the spatial refractive index profile to manipulate light in new ways to disordered assemblies of scattering particles to advance understanding of ‘random’ photonics, with a focus on lasing and nonlinear wave mixing. We are also actively exploring new photonic designs inspired by fundamental physics, such as parity-time symmetry concepts and topologically protected photonic states as new ways to protect against performance degradation by defects introduced by assembly.
RECENT ACCOMPLISHMENTS:
- Demonstrated the tuning of a random laser by controling the disorder of assembled nanowires through reconfiguration in an electric field, through collaboration between Christodoulides, Keating, and Liu.1
- Observed one-dimensional topological lasing in a Su-Schrieffer-Heeger ring resonator chain, as a result of collaboration between Rechtsman and Christodoulides.2
- Predicted and observed a topological laser, a complex semiconductor laser that achieves high robustness against disorder usin the same principles as for robust electron flow in toological insulators, via collaboration between Rechtsman and Christodoulides.3-4
- Observed first the valley Hall effect in optics, a possible route to robust reconfigurable colloidal-based photonic structures.5
- Numerically validated a metallic nanowire-enabled high-performace hybrid plasmonic waveguide showing a deep-subwavelength mode area and reasonable propagation distance.6
- Developed a solution-phase synthesis method that epitaxially seeds the growth of VO2 off of single-crystal, faceted TiO2 nanorods. The temperature of the insulator-to-metal transition of the nanocrystalline VO2 domains varies with particle size and the nature of the VO2-TiO2 interface.7
- Demonstrated highly confined sub-wavelength dielectric waveguids with a low-visibility and broadband opitical activity through tailoring the unique anisotropy and exploiting the inter-cell coupling of metasurface coatings.8
- Numerically investigated the coherent manipulation of optical chirality in the near-field of chiral metamaterials, which may improve the plasmonic nanostructure based enantiomeric sensing.9
- Observed the first Weyl points for photons in the optical frequency regime.10
- Demonstrated broadband infrared polarizers based on reconfigurable nanowire assemblies driven by electrical signals, through collaboration between Werner and Keating.11
- Demonstrated a vanadium dioxide (VO2) integrated photonic metadevice exhibiting electrically switchable reflection, programmable memory effect, and active infrared camouflage, as a result of collaboration between Mayer and Werner.12
- Realized the first all-dielectric lossless perfect magnetic mirror with a near-zero reflection phase at a wavlength of arround 1.0 µm, via collaboration between Liu, Werner, and Mayer.13
- Demonstrated the successful engineering of the optical properties of a nanoring loaded nanoantenna system at a deep-subwavelength scale, through collaboration between Werner and Mayer.14, 15
- Demonstrated the cloaking phenomena of microwave radiators through dispersion engineeing of metasurface.16
References:
1. Donahue, P. D.; Zhang, C.; Nye, N.S.; Miller, J. R.; Wang, C. -Y.; Tang, R.; Christodoulides, D. N.; Keating, C. D.; Liu, Z. Controlling Disorder by Electric Field Directed Reconfiguration of Nanowires to Tune Random Lasing. ACS Nano 2018 12, 7, 7343–7351. DOI: 10.1021/acsnano.8b03829
2. Parto, M.; Wittek, S.; Hodaei, H.; Harari, G.; Bandres, M. A.; Ren, J.; Rechtsman, M. C.; Segev, M.; Christodoulides, D. N.; Khajavikhan, M. Edge-Mode Lasing in 1D Topological Active Arrays. Phys. Rev. Lett. 2018, 120 (11), 113901. DOI: 10.1103/PhysRevLett.120.113901
3. Bandres, M. A.; Wittek, S.; Harari, G.; Parto, M.; Ren, J.; Segev, M.; Christodoulides, D. N.; Khajavikhan, M. Topological Insulator Laser: Experiments. Science 2018, 359 (6381), eaar4005. DOI: 10.1126/science.aar4005
4. Harari, G.; Bandres, M. A.; Lumer, Y.; Rechtsman, M. C.; Chong, Y. D.; Khajavikhan, M.; Christodoulides, D. N.; Segev, M. Topological Insulator Laser: Theory. Science 2018, 359 (6381), eaar4003. DOI: 10.1126/science.aar4003
5. Noh, J.; Huang, S.; Chen, K. P.; Rechtsman, M. C. Observation of Photonic Topological Valley Hall Edge States. Phys. Rev. Lett. 2018, 120 (6), 063902. DOI: 10.1103/PhysRevLett.120.063902
6. Werner, D. H.; Kang, L.; Werner, P. L.; Ren, Q.; Yue, T.; Bian, Y. Deep-Subwavelength Light Transmission in Hybrid Nanowire-Loaded Silicon Nano-Rib Waveguides. Photonics Res. 2018, 6 (1), 37–45. DOI: 10.1364/PRJ.6.000037
7. Li, X.; Schaak, R. E. Size- and Interface-Modulated Metal-Insulator Transition in Solution-Synthesized Nanoscale VO2-TiO2-VO2 Heterostructures. Angew. Chem. Int. Ed. 2017, 56 (49), 15550–15554. DOI: 10.1002/anie.201706599
8. Jiang, Z. H.; Kang, L.; Werner, D. H. Conformal Metasurface-Coated Dielectric Waveguides for Highly Confined Broadband Optical Activity with Simultaneous Low-Visibility and Reduced Crosstalk. Nat Comms 2017, 8 (1), 356. DOI: 10.1038/s41467-017-00391-0
9. Kang, L.; Ren, Q.; Werner, D. H. Leveraging Superchiral Light for Manipulation of Optical Chirality in the Near-Field of Plasmonic Metamaterials. ACS Photonics 2017, 4 (6), 1298–1305. DOI: 10.1021/acsphotonics.7b00057
10. Noh, J.; Huang, S.; Leykam, D.; Chong, Y. D.; Chen, K. P.; Rechtsman, M. C. Experimental Observation of Optical Weyl Points and Fermi Arc-Like Surface States. Nat Phys 2017, 13 (6), 611–617. DOI: 10.1038/nphys4072
11. Boehm, S. J.; Kang, L.; Werner, D. H.; Keating, C. D. Field-Switchable Broadband Polarizer Based on Reconfigurable Nanowire Assemblies. Adv. Funct. Mater. 2017, 27 (5), 1604703. DOI: 10.1002/adfm.201604703
12. Lin, L.; Jiang, Z. H.; Ma, D.; Yun, S.; Liu, Z.; Werner, D. H.; Mayer, T. S. Dielectric Nanoresonator Based Lossless Optical Perfect Magnetic Mirror with Near-Zero Reflection Phase. Appl. Phys. Lett. 2016, 108 (17), 171902. DOI: 10.1063/1.4947274
13. Dey, K. K.; Pong, F. Y.; Breffke, J.; Pavlick, R.; Hatzakis, E.; Pacheco, C.; Sen, A. Dynamic Coupling at the Ångström Scale. Angew. Chem. Int. Ed. 2015, 55 (3), 1113–1117. DOI: 10.1002/anie.201509237
14.Panaretos, A. H.; Yuwen, Y. A.; Werner, D. H.; Mayer, T. S. Tuning the Optical Response of a Dimer Nanoantenna Using Plasmonic Nanoring Loads. Sci. Rep. 2015, 5 (1), 9813. DOI: 10.1038/srep09813
15. Panaretos, A. H.; Yuwen, Y. A.; Werner, D. H.; Mayer, T. S. Corrigendum: Tuning the Optical Response of a Dimer Nanoantenna Using Plasmonic Nanoring Loads. Sci. Rep. 2016, 6 (1), 21942. DOI: 10.1038/srep21942
16. Jiang, Z. H.; Werner, D. H. Dispersion Engineering of Metasurfaces for Dual-Frequency Quasi-Three-Dimensional Cloaking of Microwave Radiators. Opt. Express 2016, 24 (9), 9629–9644. DOI: 10.1364/OE.24.009629